A seasoned deer hunter is shocked when his hound dog trots up with a human femur clenched between its teeth. A woman veers off her normal urban walking path and happens upon a human skull. New property owners commission a land survey that reveals a set
Forget dystopian scenarios – AI is pervasive today, and the risks are often hidden
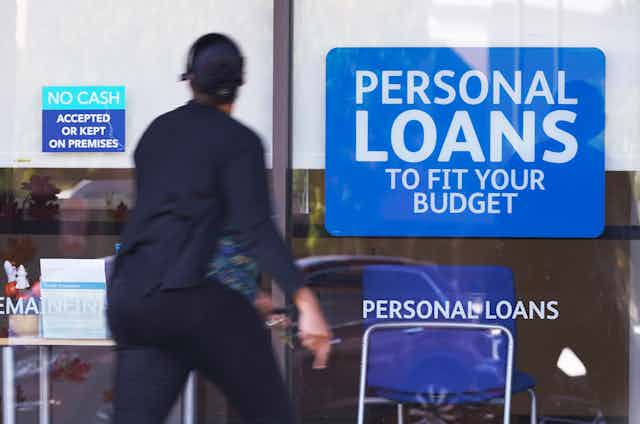
The turmoil at ChatGPT-maker OpenAI, sparked by the board of directors firing high-profile CEO Sam Altman[1] on Nov. 17, 2023, has put a spotlight on artificial intelligence safety and concerns about the rapid development of artificial general intelligence, or
How do viruses get into cells? Their infection tactics determine whether they can jump species or set off a pandemic
COVID-19, flu, mpox, noroviral diarrhea: How do the viruses that cause these diseases actually infect you?
Viruses cannot replicate on their own[1], so they must infect cells in your body to make more copies of themselves. The life cycle of a virus can
Thank gluten's complex chemistry for your light, fluffy baked goods
Within the bread, rolls and baked goods on many tables this holiday season is an extraordinary substance – gluten. Gluten’s unique chemistry makes foods airy and stretchy.
I’m a chemist[1] who teaches a chemistry of cooking class, and every year I ask
Read more https://theconversation.com/thank-glutens-complex-chemistry-for-your-light-fluffy-baked-goods-216869
Pooling multiple models during COVID-19 pandemic provided more reliable projections about an uncertain future
How can anyone decide on the best course of action in a world full of unknowns?
There are few better examples of this challenge than the COVID-19 pandemic, when officials fervently compared potential outcomes as they weighed options like whether to implement
Every state is about to dole out federal funding for broadband internet – not every state is ready for the task
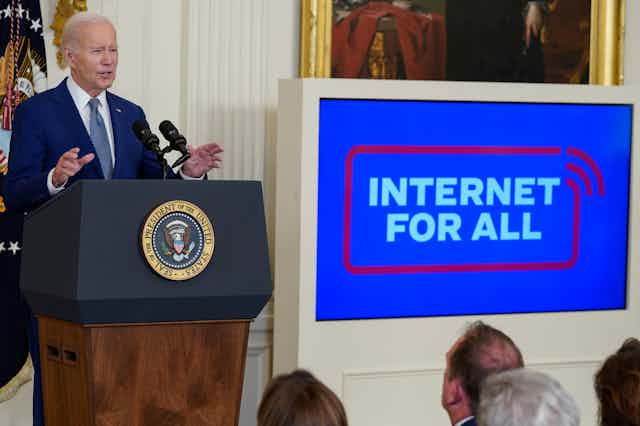
When the Infrastructure Investment and Jobs Act was signed in late 2021, it included US$42.5 billion for broadband[1] internet access as part of the Broadband Equity, Access, and Deployment Program. The program aims to ensure that broadband access is
Forget ‘Man the Hunter’ – physiological and archaeological evidence rewrites assumptions about a gendered division of labor in prehistoric times
Prehistoric men hunted; prehistoric women gathered. At least this is the standard narrative written by and about men to the exclusion of women.
The idea of “Man the Hunter” runs deep within anthropology, convincing people that hunting made us human, only