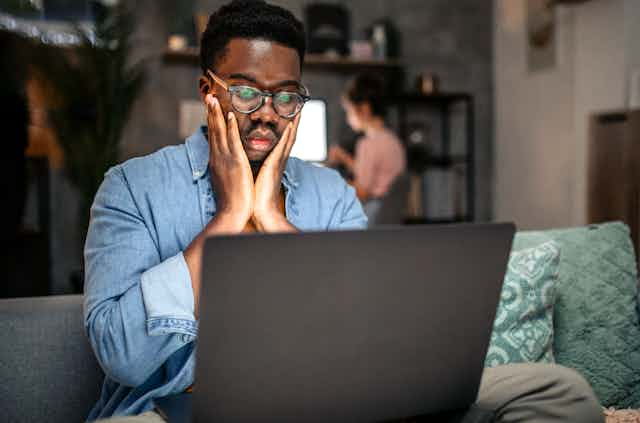
Information warfare abounds, and everyone online has been drafted[1] whether they know it or not.
Disinformation is deliberately generated misleading content disseminated for selfish or malicious purposes. Unlike misinformation, which may be shared unwittingly or