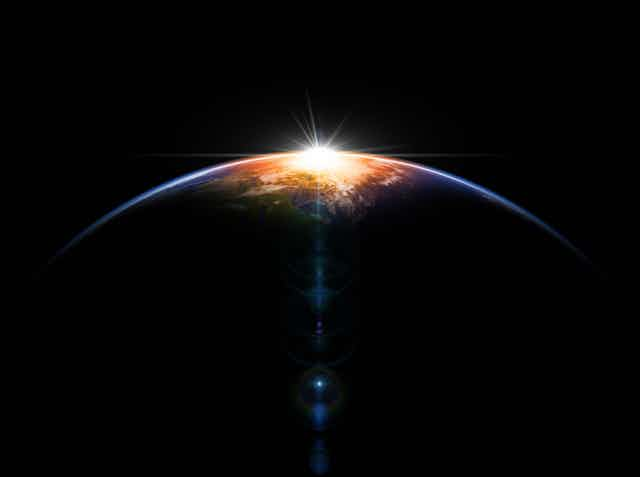
For many years, scientists have predicted that many of the elements that are crucial ingredients for life[1], like sulfur and nitrogen, first came to Earth when asteroid-type objects carrying them crashed into our planet’s surface.
For many years, scientists have predicted that many of the elements that are crucial ingredients for life[1], like sulfur and nitrogen, first came to Earth when asteroid-type objects carrying them crashed into our planet’s surface.
When most people hear about electroconvulsive therapy, or ECT[1], it typically conjures terrifying images of cruel, outdated and pseudo-medical procedures. Formerly known as electroshock therapy, this perception of ECT as dangerous and ineffective has been
The virtue of intellectual humility is getting a lot of attention. It’s heralded as a part of wisdom[1], an aid to self-improvement[2] and a catalyst for more productive political dialogue[3]. While researchers define intellectual humility in various ways,
Read more https://theconversation.com/intellectual-humility-is-a-key-ingredient-for-scientific-progress-211410
Dec. 10 marks the anniversary of the 1948 signing of the Universal Declaration of Human Rights[1], adopted by the United Nations[2] in the aftermath of the Holocaust. Though contested, imperfect and unfulfilled, the declaration remains a milestone in human
If you hadn’t heard about superconductors before 2023, odds are you know what they are now. Researchers raised eyebrows early in the year with claims of operational room-temperature superconductors[1], though none has been substantiated, and one paper[2] from
The rampant increase of hate messages[1] on social media is a scourge in today’s technology-infused society. Racism, homophobia, xenophobia and even personal attacks on people who have the audacity to disagree[2] with someone else’s political opinion –